

Golang runtime内存管理机制
source link: https://longzhiri.github.io/Golang+Runtime+Memory+Management.html
Go to the source link to view the article. You can view the picture content, updated content and better typesetting reading experience. If the link is broken, please click the button below to view the snapshot at that time.
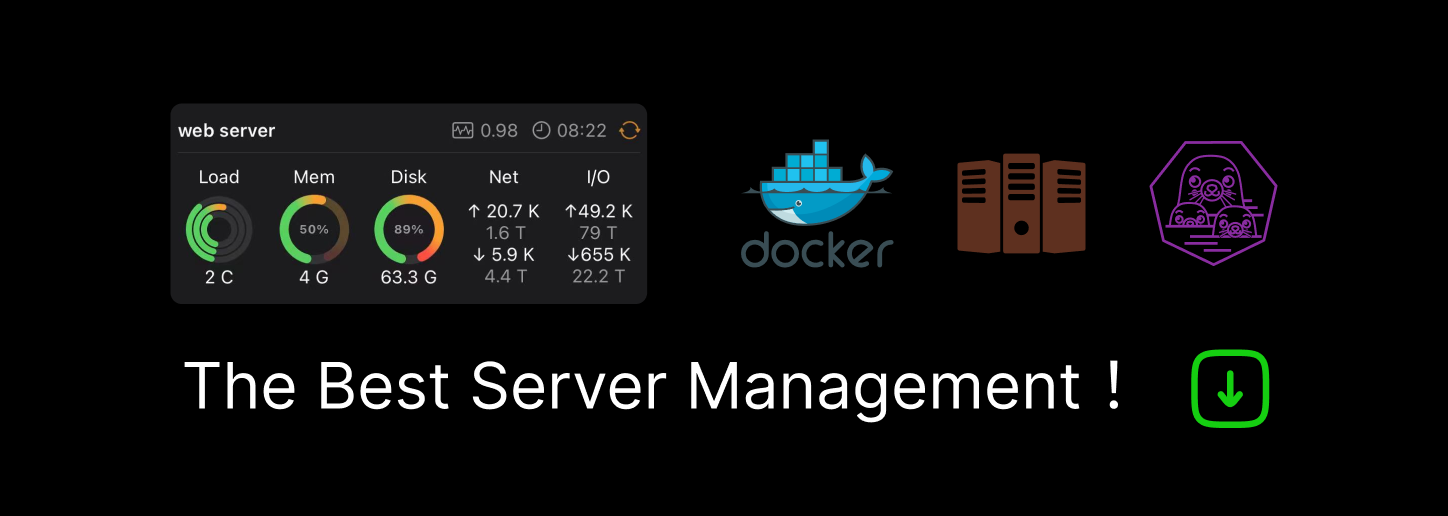
Golang runtime内存管理机制
工作中遇到一个golang项目内存占用非常多,于是根据pprof工具反馈的热点做了优化,优化后通过pprof能看出来改善明显,原来的热点几乎不见了,但是top上显示的RSS内存占用却没什么变化,于是用runtime.ReadMemStats想看下runtime自己统计的内存占用相关信息,这个函数返回的结构体MemStats:
type MemStats struct {
// Alloc is bytes of allocated heap objects.
//
// This is the same as HeapAlloc (see below).
Alloc uint64
// TotalAlloc is cumulative bytes allocated for heap objects.
//
// TotalAlloc increases as heap objects are allocated, but
// unlike Alloc and HeapAlloc, it does not decrease when
// objects are freed.
TotalAlloc uint64
// Sys is the total bytes of memory obtained from the OS.
//
// Sys is the sum of the XSys fields below. Sys measures the
// virtual address space reserved by the Go runtime for the
// heap, stacks, and other internal data structures. It's
// likely that not all of the virtual address space is backed
// by physical memory at any given moment, though in general
// it all was at some point.
Sys uint64
// Lookups is the number of pointer lookups performed by the
// runtime.
//
// This is primarily useful for debugging runtime internals.
Lookups uint64
// Mallocs is the cumulative count of heap objects allocated.
// The number of live objects is Mallocs - Frees.
Mallocs uint64
// Frees is the cumulative count of heap objects freed.
Frees uint64
// HeapAlloc is bytes of allocated heap objects.
//
// "Allocated" heap objects include all reachable objects, as
// well as unreachable objects that the garbage collector has
// not yet freed. Specifically, HeapAlloc increases as heap
// objects are allocated and decreases as the heap is swept
// and unreachable objects are freed. Sweeping occurs
// incrementally between GC cycles, so these two processes
// occur simultaneously, and as a result HeapAlloc tends to
// change smoothly (in contrast with the sawtooth that is
// typical of stop-the-world garbage collectors).
HeapAlloc uint64
// HeapSys is bytes of heap memory obtained from the OS.
//
// HeapSys measures the amount of virtual address space
// reserved for the heap. This includes virtual address space
// that has been reserved but not yet used, which consumes no
// physical memory, but tends to be small, as well as virtual
// address space for which the physical memory has been
// returned to the OS after it became unused (see HeapReleased
// for a measure of the latter).
//
// HeapSys estimates the largest size the heap has had.
HeapSys uint64
// HeapIdle is bytes in idle (unused) spans.
//
// Idle spans have no objects in them. These spans could be
// (and may already have been) returned to the OS, or they can
// be reused for heap allocations, or they can be reused as
// stack memory.
//
// HeapIdle minus HeapReleased estimates the amount of memory
// that could be returned to the OS, but is being retained by
// the runtime so it can grow the heap without requesting more
// memory from the OS. If this difference is significantly
// larger than the heap size, it indicates there was a recent
// transient spike in live heap size.
HeapIdle uint64
// HeapInuse is bytes in in-use spans.
//
// In-use spans have at least one object in them. These spans
// can only be used for other objects of roughly the same
// size.
//
// HeapInuse minus HeapAlloc estimates the amount of memory
// that has been dedicated to particular size classes, but is
// not currently being used. This is an upper bound on
// fragmentation, but in general this memory can be reused
// efficiently.
HeapInuse uint64
// HeapReleased is bytes of physical memory returned to the OS.
//
// This counts heap memory from idle spans that was returned
// to the OS and has not yet been reacquired for the heap.
HeapReleased uint64
// HeapObjects is the number of allocated heap objects.
//
// Like HeapAlloc, this increases as objects are allocated and
// decreases as the heap is swept and unreachable objects are
// freed.
HeapObjects uint64
// StackInuse is bytes in stack spans.
//
// In-use stack spans have at least one stack in them. These
// spans can only be used for other stacks of the same size.
//
// There is no StackIdle because unused stack spans are
// returned to the heap (and hence counted toward HeapIdle).
StackInuse uint64
// StackSys is bytes of stack memory obtained from the OS.
//
// StackSys is StackInuse, plus any memory obtained directly
// from the OS for OS thread stacks (which should be minimal).
StackSys uint64
// MSpanInuse is bytes of allocated mspan structures.
MSpanInuse uint64
// MSpanSys is bytes of memory obtained from the OS for mspan
// structures.
MSpanSys uint64
// MCacheInuse is bytes of allocated mcache structures.
MCacheInuse uint64
// MCacheSys is bytes of memory obtained from the OS for
// mcache structures.
MCacheSys uint64
// BuckHashSys is bytes of memory in profiling bucket hash tables.
BuckHashSys uint64
// GCSys is bytes of memory in garbage collection metadata.
GCSys uint64 // Go 1.2
// OtherSys is bytes of memory in miscellaneous off-heap
// runtime allocations.
OtherSys uint64 // Go 1.2
// NextGC is the target heap size of the next GC cycle.
//
// The garbage collector's goal is to keep HeapAlloc ≤ NextGC.
// At the end of each GC cycle, the target for the next cycle
// is computed based on the amount of reachable data and the
// value of GOGC.
NextGC uint64
// LastGC is the time the last garbage collection finished, as
// nanoseconds since 1970 (the UNIX epoch).
LastGC uint64
// PauseTotalNs is the cumulative nanoseconds in GC
// stop-the-world pauses since the program started.
//
// During a stop-the-world pause, all goroutines are paused
// and only the garbage collector can run.
PauseTotalNs uint64
// PauseNs is a circular buffer of recent GC stop-the-world
// pause times in nanoseconds.
//
// The most recent pause is at PauseNs[(NumGC+255)%256]. In
// general, PauseNs[N%256] records the time paused in the most
// recent N%256th GC cycle. There may be multiple pauses per
// GC cycle; this is the sum of all pauses during a cycle.
PauseNs [256]uint64
// PauseEnd is a circular buffer of recent GC pause end times,
// as nanoseconds since 1970 (the UNIX epoch).
//
// This buffer is filled the same way as PauseNs. There may be
// multiple pauses per GC cycle; this records the end of the
// last pause in a cycle.
PauseEnd [256]uint64 // Go 1.4
// NumGC is the number of completed GC cycles.
NumGC uint32
// NumForcedGC is the number of GC cycles that were forced by
// the application calling the GC function.
NumForcedGC uint32 // Go 1.8
// GCCPUFraction is the fraction of this program's available
// CPU time used by the GC since the program started.
//
// GCCPUFraction is expressed as a number between 0 and 1,
// where 0 means GC has consumed none of this program's CPU. A
// program's available CPU time is defined as the integral of
// GOMAXPROCS since the program started. That is, if
// GOMAXPROCS is 2 and a program has been running for 10
// seconds, its "available CPU" is 20 seconds. GCCPUFraction
// does not include CPU time used for write barrier activity.
//
// This is the same as the fraction of CPU reported by
// GODEBUG=gctrace=1.
GCCPUFraction float64 // Go 1.5
// EnableGC indicates that GC is enabled. It is always true,
// even if GOGC=off.
EnableGC bool
// DebugGC is currently unused.
DebugGC bool
// BySize reports per-size class allocation statistics.
//
// BySize[N] gives statistics for allocations of size S where
// BySize[N-1].Size < S ≤ BySize[N].Size.
//
// This does not report allocations larger than BySize[60].Size.
BySize [61]struct {
// Size is the maximum byte size of an object in this
// size class.
Size uint32
// Mallocs is the cumulative count of heap objects
// allocated in this size class. The cumulative bytes
// of allocation is Size*Mallocs. The number of live
// objects in this size class is Mallocs - Frees.
Mallocs uint64
// Frees is the cumulative count of heap objects freed
// in this size class.
Frees uint64
}
}
尽管文档有比较详细的描述,但是有些字段理解起来依然很模糊,需要结合Golang runtime的内存管理机制来理解,本文就以此契机来深入了解下Golang1.13的内存管理。
几个重要的数据结构
mspan
Golang的内存管理是基于google tcmalloc的实现,会把不同size划分成一些size class,内存分配是根据需要的size找到最小能满足的size class,而mspan简单来的说是数页(虚拟页,大小是8KB)内存空间,根据指定的size class拆分成可用的大小相等的obj等待分配。 所有size class:
// class bytes/obj bytes/span objects tail waste max waste
// 1 8 8192 1024 0 87.50%
// 2 16 8192 512 0 43.75%
// 3 32 8192 256 0 46.88%
// 4 48 8192 170 32 31.52%
// 5 64 8192 128 0 23.44%
// 6 80 8192 102 32 19.07%
// 7 96 8192 85 32 15.95%
// 8 112 8192 73 16 13.56%
// 9 128 8192 64 0 11.72%
// 10 144 8192 56 128 11.82%
// 11 160 8192 51 32 9.73%
// 12 176 8192 46 96 9.59%
// 13 192 8192 42 128 9.25%
// 14 208 8192 39 80 8.12%
// 15 224 8192 36 128 8.15%
// 16 240 8192 34 32 6.62%
// 17 256 8192 32 0 5.86%
// 18 288 8192 28 128 12.16%
// 19 320 8192 25 192 11.80%
// 20 352 8192 23 96 9.88%
// 21 384 8192 21 128 9.51%
// 22 416 8192 19 288 10.71%
// 23 448 8192 18 128 8.37%
// 24 480 8192 17 32 6.82%
// 25 512 8192 16 0 6.05%
// 26 576 8192 14 128 12.33%
// 27 640 8192 12 512 15.48%
// 28 704 8192 11 448 13.93%
// 29 768 8192 10 512 13.94%
// 30 896 8192 9 128 15.52%
// 31 1024 8192 8 0 12.40%
// 32 1152 8192 7 128 12.41%
// 33 1280 8192 6 512 15.55%
// 34 1408 16384 11 896 14.00%
// 35 1536 8192 5 512 14.00%
// 36 1792 16384 9 256 15.57%
// 37 2048 8192 4 0 12.45%
// 38 2304 16384 7 256 12.46%
// 39 2688 8192 3 128 15.59%
// 40 3072 24576 8 0 12.47%
// 41 3200 16384 5 384 6.22%
// 42 3456 24576 7 384 8.83%
// 43 4096 8192 2 0 15.60%
// 44 4864 24576 5 256 16.65%
// 45 5376 16384 3 256 10.92%
// 46 6144 24576 4 0 12.48%
// 47 6528 32768 5 128 6.23%
// 48 6784 40960 6 256 4.36%
// 49 6912 49152 7 768 3.37%
// 50 8192 8192 1 0 15.61%
// 51 9472 57344 6 512 14.28%
// 52 9728 49152 5 512 3.64%
// 53 10240 40960 4 0 4.99%
// 54 10880 32768 3 128 6.24%
// 55 12288 24576 2 0 11.45%
// 56 13568 40960 3 256 9.99%
// 57 14336 57344 4 0 5.35%
// 58 16384 16384 1 0 12.49%
// 59 18432 73728 4 0 11.11%
// 60 19072 57344 3 128 3.57%
// 61 20480 40960 2 0 6.87%
// 62 21760 65536 3 256 6.25%
// 63 24576 24576 1 0 11.45%
// 64 27264 81920 3 128 10.00%
// 65 28672 57344 2 0 4.91%
// 66 32768 32768 1 0 12.50%
size class 对应mspan:
实际上mspan不仅是按照size class来分类,还会按照obj是否noscan来分类,size class + noscan作为key,叫做span class。这样做是为了提高gc效率,因为noscan的mspan可以不用扫描。
func makeSpanClass(sizeclass uint8, noscan bool) spanClass {
return spanClass(sizeclass<<1) | spanClass(bool2int(noscan))
}
mspan.allocBits是一个位图,标记是否某个obj已分配,每个obj index对应一位,1表示已分配,0表示未分配,真正分配的时候从mspan.freeindex开始遍历,直到找到第一个未分配的obj返回,其中为了提高效率做了些优化,比如mspan.allocCache是最近使用的allocBits的8字节cache:
mspan中其他几个常用字段的意思:
allocCount:已经分配了多少个obj;
nelems:总共有多少个obj;
startAddr:mspan中管理的内存的起始地址,用于计算obj的地址;
npages:mspan中总共管理多少页内存;
mcache
mcache可以认为是每个P(Go的虚拟核心)私有的内存cache,不与其他P共享,因此访问不需要加锁,效率最高。它包含:
- alloc: span class到mspan的映射map;
- tiny, tinyoffset: 微小内存(<=16字节)管理;
在mcache中的分配流程(可见malloc.go:mallocgc的实现)按照分配的size会有不同的逻辑:
- 微小内存分配:size<=16字节,且分配的对象不包含指针(gc时不需要扫描)会根据当前tinyoffset判定tiny中是否有足够内存分配,如果有则直接切走返回,如果没有则去size class为16字节的mspan中分配一个空闲obj,从obj中切走指定size,如果还有剩余则cache到tiny中以备下次使用;
- 小内存分配:size<=32KB,先根据size找到对应的size class,再通过alloc映射表找到对应的mspan,从mspan中分配出一个空闲的obj,如果这个mspan不存在任何空闲的obj,会往下层请求分配一个可用的mspan(这个后文会描述)放到mcache中替换老的mspan,然后用新的mspan分配一个空闲obj;
- 大内存分配:直接从下层分配一个全新的mspan,取第一个obj返回,注意这个mspan并不缓存到mcache中;
我们必须关注的一些细节是:
- mallocgc函数返回的内存往往会比请求的内存要多,这个一个是因为分配的内存地址需要对齐,一个是因为mspan中的obj都是等大小的内存块。
mcentral
mcentral管理同类mspan(span class相同),在多个P之间共享,对其操作需要加锁。mcentral结构主要由两个mspan list构成:
- nonempty: 存放还有可分配obj的mspan;
- empty:存放已经被cache到各个P的mcache中的mspan,也就是不能再分配的mspan;
在上一节mcache分配内存的步骤2中我们讲到,当某个mspan没有任何空闲obj可分配,则会去下层请求,这个请求其实转到了对应的mcentral的cacheSpan函数,这个函数的流程大致是从nonempty摘除一个mspan,放到empty中去,并返回给对应mcache让其使用,还有部分逻辑是在一边触发gc的sweep操作释放obj,因为即便在empty list中的span可能还未sweep,说不定sweep后也能空出可用的span。
我们必须关注的细节是:mcentral管理的内存,对应的是small size classes,也就是上节中的第二种情况。
mheap
mheap属于内存分配的最底层,主要有三个字段:
- central:所有不同span class到对应mcentra的l映射map;
- free:是一个Treap数据结构,所有free span都存放在此,每一个Treap node都存放一个free span;
- arenas:heapArena map,实际是数组,使用arenaIndex做索引来访问;
heap arena
Go把堆空间划分成一块块等大小的arena,叫做heap arena,所有span都是在arena上分配,在64bit系统上这个大小是64MB,每个heap arena的起始地址都是和size对齐的,并且分配器尽量保证arena之间内存连续。数据结构heapArena对应一块arena的元数据,mheap.arenas对所有arena做两层数组索引,两层索引分别叫做L1和L2,在64bit系统上L1为0,可以认为只有L2这层索引。任何地址如果需要找到对应的heapArena,需要先转化为arenaIndex,其实就是算出L1和L2索引:
func arenaIndex(p uintptr) arenaIdx {
return arenaIdx((p - arenaBaseOffset) / heapArenaBytes)
}
type arenaIdx uint
func (i arenaIdx) l1() uint {
if arenaL1Bits == 0 {
// Let the compiler optimize this away if there's no
// L1 map.
return 0
} else {
return uint(i) >> arenaL1Shift
}
}
func (i arenaIdx) l2() uint {
if arenaL1Bits == 0 {
return uint(i)
} else {
return uint(i) & (1<<arenaL2Bits - 1)
}
}
heapArenaBytes: arena区域的大小; arenaBaseOffset:这个偏移值是因为在amd64中,其实只用了48位地址,高16位虽然没用到,但是需要符号扩展,比如第48位为1的地址,高16位也得是1,所以你得减去一个偏移值0xffff800000000000才能抵消这部分符号扩展;
heap arena map 图示:
heap arena的元数据:
- bitmap [heapArenaBitmapBytes]byte: 表示这块arena中每指针大小的内存块是否是指针,这个主要是用来给gc扫描用,大致用途是gc标记扫描一个obj时,会按照指针大小步进(假设obj有字段是指针,偏移值必须是按照指针大小对齐的,所以按指针大小步进肯定能遍历到这个指针字段),并假设这个字段是指针,然后将字段的地址映射到bitmap中的位信息来确认,如果是指针,需要把这个字段当作新的obj放入工作队列等待下次扫描(三色标记中的置成灰色)。其实bitmap中用两位表示一个指针大小内存,具体细节如果以后讲gc可以详细描述。这个位信息是在malloc分配内存的时候由传进来的type类型信息来设置;
- spans [pagesPerArena]*mspan: 表示arena中每一页对应在哪个span中,大致用途是先求出某个地址所在heap arena,再求出所在这个arena的哪一页,再根据这个map得到属于哪个mspan,进而求出在这个mspan的哪个obj中,比如gc扫描一个obj发现有一个字段是指针,用这种方式就能得到这个指针所指向的obj;
heap arena 元数据图示:
mheap中的内存分配
上接mcentral中的内存分配流程,在mcentral.cacheSpan函数中,如果取不到任何可用的span,会把请求转发到mheap这层,mheap.alloc函数负责分配指定页大小的全新span,主要流程如下:
- 先从free树中遍历找到一个满足指定页大小的span,由于free树是按照地址大小有序,查找方式是中序遍历,将会找到一个最小地址的满足size大于等于指定页的span;
- 如果步骤1没有找到合适的span,mheap会走到grow函数,通过向系统申请内存(sysAlloc)补充free,再重复步骤1;
- 最后如果找到的span 页数大于需求的页数,需要新创建一个指定页数的span返回,并把free树中的目标span减去指定页数,剩余的以备后用;
这里有必要详细了解下sysAlloc向系统申请内存。在Go中把内存区域标为了4个状态:
- None: 默认初始状态;
- Reserved:指的是被runtime预留的内存,但是还不能直接访问,对应的函数调用是sysReserve,在linux系统中的实现是通过mmap设置prot参数为PROT_NONE;
- Prepared: 还是不能直接访问,但是可以快速转化到Ready状态,对应的函数调用是sysMap,在linux系统中其实是再次调用mmap设置prot参数为可读写了,理论上已经能访问了,windows上这个函数没有实际功能;
- Ready:可以直接访问,对应的函数调用是sysUsed,linux上没有实际逻辑,windows上是commit memory;
sysAlloc分配一个或多个heap arena空间,大小要大于其参数传进来的字节数,分配过程是先调用sysReserve向系统申请保留指定内存空间,再调用sysMap把状态转为Prepared状态,同时为新分配的heap arena空间创建metadata,最后返回Prepared状态的内存首地址。
在grow的流程中新分配的内存空间会尝试和在地址空间上处于前后的span合并,然后再插入进free树中。
Go程序中使用的内存主要是在GC时释放的,具体来说主要有下面几个释放流程:
- 在GC的mark termination阶段,每个P的mcache会清空,对应的mcentral中的span会从empty list放入nonempty list中成为可用状态;
- 在GC的sweep阶段,会sweep各个unswept spans,如果这个span中还有已分配的obj在被引用,但是仍有obj未分配,确保放入对应mcentral的nonempy list中以备后用,如果这个span都空了,整个span都需要释放,可以释放到更下层mheap中去,确切的说是放到mheap中的free树中,同时释放的时候也会尝试和在地址空间上邻近的span合并。sweep过程是在独立后台goroutine中持续进行,直到下个GC周期开始时在执行sweep termination流程后结束(具体可见函数调用gcStart->finishsweep_m);
- 后台scavenger goroutine根据一定策略每隔一段时间清扫一定内存,这部分内存会直接释放给系统,不像1,2两种释放其实内存还是在runtime的堆中,具体来说调用了sysUnused,这个函数在linux中的实现是以参数DONTNEED调用madvise,并不是说内存立即释放,在top中也不一定能立即看到RSS的下降,这个仅是一个advise,通知系统支持这块内存区域的物理页不需要了,系统有自己的策略去回收。runtime/debug中的FreeOSMemory函数可以主动触发一次这个释放过程,并且是尽可能释放free树中的空闲内存;
可以看出来释放过程是分配过程的逆过程,内存分配时从系统流转过来,在不同层级cache,释放时又会释放不同层级的cache,最终流转回系统。为什么内存有这么多cache,主要是为了分配效率更高,类似CPU的L1/L2/L3 cache原理。
有一个值得注意的细节是:在free树中的span内存有可能是非ready状态,也就是从free树中分配下来的span可能需要调用sysUsed变成ready状态才能使用,这个是根据mspan.scavenged来判断的。具体原因是从系统刚分配出来的内存和scavenger释放给系统的内存状态(虽然释放了但还是作为scavenged的mspan在free树中)都是非ready状态。
runtime内存统计
回到文章开头的问题,runtime本身有非常详细的内存各种数据统计,在阐述了整个内存管理机制后,我们可以更深入理解MemStats结构中一些字段代表的具体意义了。
- HeapAlloc:已经分配的obj总字节数,包括被引用着的obj和还没来得及被GC清理的未被引用的obj。大致计算方式是,首先统计历史总共分配了多少字节,mcentral记录了这个spanClass已经分配了多少个obj,乘以对应size然后累加起来,这种方式会把cache到mcache中的mspan的所有obj统计到这个分配总数中,但是其实按照我们前文所述并不是所有obj都被分配了,然后统计历史总共释放了多少字节(gc sweep总共free了多少字节),span的sweep流程中会把释放obj个数统计到mcache.local_nsmallfree的对应sizeClass中,在gc mark termination阶段再统计到代表历史总释放的mheap.nsmallfree中,最后历史总分配减去历史总释放即得到HeapAlloc;
- HeapSys: 向系统申请分配的虚拟内存地址总空间,包括那些可能背后没有映射到物理内存的空间,主要是在sysAlloc中调用sysMap增长,注意即便scavenger调用sysUnused也不会减少这个值;
- HeapIdle:包括所有空闲的span,没有分配任何obj,放在mheap.free树中,mheap分配span的时候会降低这个值;
- HeapInuse: 包括所有正在使用的span,也即只要有一个obj被分配了,这个span都会被记录,这个字段和HeapAlloc的区别是,HeapInuse还包含那些还未分配给mcache的span,可以认为统计的是mcentral中所有的span;
- HeapReleased: 返还给OS的物理内存,就是上文中所述的scavenger调用sysUnused的内存,当然分配的Prepared状态的内存也会预先算在HeapReleased中,等待变成Ready状态被使用再被减去;
一些值得注意的细节:
- 在runtime中使用HeapSys-HeapReleased代表对RSS的估计;
- 设置环境变量GODEBUG=’gctrace=1’会每隔一段时间打印scavenger工作时的一些统计日志,包括HeapSys,HeapIdle,HeapInuse,HeapReleased字段,如下:
Memory Profiler 原理
在Go中对内存做性能分析,一般会用到pprof工具,比如在import “net/http/pprof”并启动一个http server后,就可以如下方式获得性能分析结果:
go tool pprof http://localhost:6060/debug/pprof/heap
但是通常在profile中显示的内存占用不仅与系统中显示的RSS差距很大,还和runtime.MemStats中显示的内存占用差距较大,这主要原因是Memory Profiler的机制是在分配内存时进行采样,并不是说每一次mallocgc调用都会触发一次采样,当然这个采样频率是受一个变量控制的,MemProfileRate控制采样频率,设置为1会导致每次分配都会触发采样,实测非常影响性能,几乎程序跑不起来,默认值是512*1024,可以大致认为是每512K采一次样,实际是更精细复杂的控制。可以通过设置环境变量GODEBUG=’memprofilerate=1’来控制变量值为1。
Memory Profiler统计内存分配是实时统计,但是由于内存释放是在gc sweep阶段才释放,不是说某个obj程序说不用了会立即可以释放,如果我们对内存占用做实时快照肯定会得到内存占用偏高的结论。因为刚分配的内存最快释放的时机是在下一次gc mark termination之后gc sweep持续工作的一段时间里的某一个时刻里面发生,所以profiler以gc mark termination时机作为时间参考坐标,这之前分配的内存和这之后下次gc前发生的内存释放处于一个快照中进行内存统计。
具体实现是分配的时候以调用堆栈的各个函数的pc地址作hash,到一个hash表中索引一个bucket(带调用堆栈pc列表),记录此次分配字节数,并且在span上记录这个分配的obj对应的bucket,当obj在gc sweep释放时,找到对应的bucket记录此次释放,同时同一个bucket中的内存分配和释放的记录要按照同一个快照匹配记录,不能是这个周期的释放统计到另一个周期里面去了。在每一个gc mark termination时都会把当前快照的统计记录到一个历史总的(active)统计里面去,外面接口获取的当前memory profile,即获得的是这个active统计,反应的是当前最新的内存使用情况。
可以看出来Go的堆内存管理主要是分层管理,类似CPU的多级cache,最近的访问效率越高,资源越少越贵,算法有些类似常用的Buddy和Slab算法,同时对多核多线程用况做了优化,总体来说设计简单清晰,复杂度多是GC带入的,如果没有GC就像C++ tcmalloc,实现会更加精简。
Recommend
About Joyk
Aggregate valuable and interesting links.
Joyk means Joy of geeK